Projects
Using bifluxon tunneling to protected the Fluxonium qubit
In this project, we investigate a process of bi-fluxon tunneling in the Fluxonium qubits.
First, the Fluxonium qubit typically consists of a superconducting loop (inductance) interrupted by a Josephson junction. This configuration renders the qubit highly sensitive to external magnetic flux, thereby allowing manipulation of its energy level structure using this flux. Fluxonium qubits are often operated at what is known as the half-integer flux quantum. At this point, the system attains a state of symmetry. The potential energy landscape of the Fluxonium becomes symmetric, which offers several operational advantages. For instance, this symmetry leads to first order insensitivity to flux noise, which is major sources of dephasing in flux qubit.
Furthermore, at the half flux quantum, the energy levels of the qubit may exhibit avoided crossings, resulting in a non-trivial energy gap. This gap, typically characterized by low frequency, contributes to a small charge dipole moment, which aids in isolating the qubit from environmental noise.
Our goal is to create a Fluxonium qubit working at zero external flux, with a key goal of designing a circuit featuring substantially increased superinductance. This enhancement in the Fluxonium's inductance resulted in a complex, multi-welled potential landscape at zero external flux. In this unique landscape, we strategically positioned the ground state of the qubit in the lowest potential well, while distributing the excited state across two adjacent wells.
This design played a pivotal role in minimizing the overlap between quantum states, thereby providing a stable operational foundation for bi-fluxon tunneling at zero external flux. We use the term 'bi-fluxon' to describe this phenomenon because, unlike a single fluxon transition which involves tunneling from one well to an adjacent well, our design involves tunneling across two next-neighboring wells. This innovative approach allowed us to encode quantum information into states with distinct fluxon parities, culminating in a high-coherence qubit that operates at higher frequencies. Such an advantage is significant, as it enables us to apply methodologies developed for transmon qubits to our more coherent Fluxonium design.
For more details about this project please check our publications!
Realizing a hyperinductance Fluxonium.
Constructing super-inductances is already a complex task, and developing what we term 'hyper-inductances' presents even greater challenges.
One of the primary hurdles is the inherent design of superinductance, which involves a large array of substantial Josephson junctions. Each junction, being a superconductor on a semiconductor substrate, has an inevitable stray capacitance to the ground. This results in an inductor with additional, unintended capacitance, leading to the creation of unwanted 'chain modes.' These modes have a strong coupling effect on the Fluxonium, acting as a noise channel and adversely affecting qubit performance.
To address the issue of chain modes, a novel approach involves etching the semiconductor silicon material surrounding the chain. This technique is expected to reduce stray capacitance, as the capacitance of the electric field in silicon is higher compared to that in vacuum due to different permeability.
However, this etching process adds significant complexity to the already intricate task of building high superinductances.
Achieving higher values of inductance opens doors to numerous applications, not just in the realm of Fluxonium but also in other areas like the development of protected 0-π qubit and various experimental research topics.
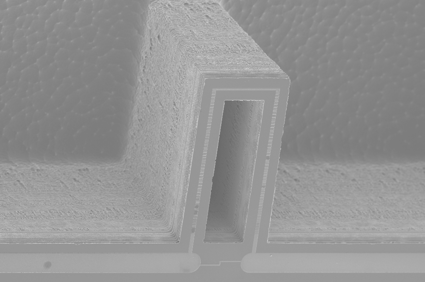
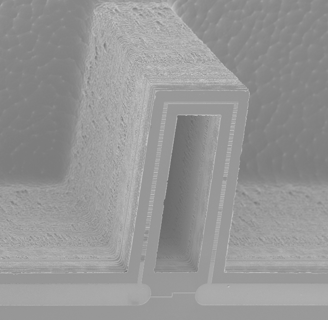